

Previous molecular approaches to determine the Phillips catalyst ethylene polymerization mechanism focused on systems containing preformed Cr–C bonds ( 5– 7). The low number of active sites is one of the main limiting factors in using spectroscopic methods to study this material because the spectroscopic signature mainly belongs to inactive species.
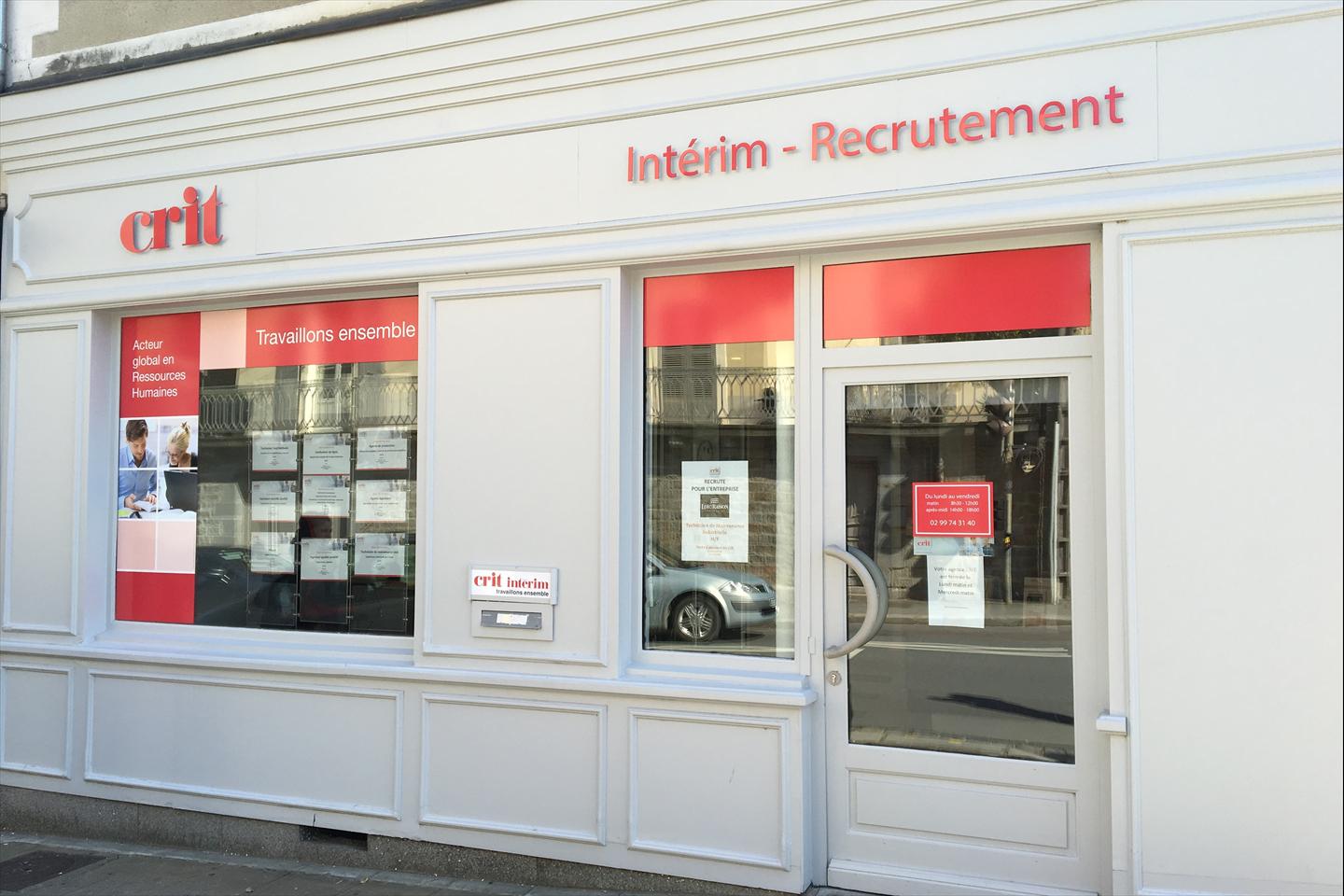

Numerous spectroscopic techniques established that the Phillips catalyst contains a complex mixture of surface Cr species, of which only ∼10% are active in polymerization ( 3, 4). Despite 50 y of research, the catalytically active site and the initiation mechanism, particularly the formation of the first Cr–C bond, remain controversial. The Phillips catalyst is active in the absence of activators that are typically required for polymerization catalysts ( 2). Contacting this material with ethylene forms the active reduced species in situ that polymerizes ethylene. This catalyst is prepared by incipient wetness impregnation of a chromium salt on silica, followed by high temperature calcination. From IR spectroscopy and DFT calculations, these tricoordinated Cr(III) sites initiate and regulate the polymer chain length via unique proton transfer steps in polymerization catalysis.Īlmost half of the world’s high-density polyethylene is produced by the Phillips catalyst, a silica-supported chromium oxide (CrO x/SiO 2) ( 1). Density functional theory (DFT) calculations using cluster models show that active sites are tricoordinated Cr(III) centers and that the presence of an additional siloxane bridge coordinated to Cr leads to inactive species. CO binding, EPR spectroscopy, and poisoning studies indicate that two different types of Cr(III) sites are present on the surface, one of which is active in polymerization. These sites are highly active in ethylene polymerization to yield polyethylene with a broad molecular weight distribution, similar to that typically obtained from the Phillips catalyst. Mononuclear Cr(III) surface sites were synthesized from grafting on silica partially dehydroxylated at 700 ☌, followed by a thermal treatment under vacuum, and characterized by infrared, ultraviolet-visible, electron paramagnetic resonance (EPR), and X-ray absorption spectroscopy (XAS).
